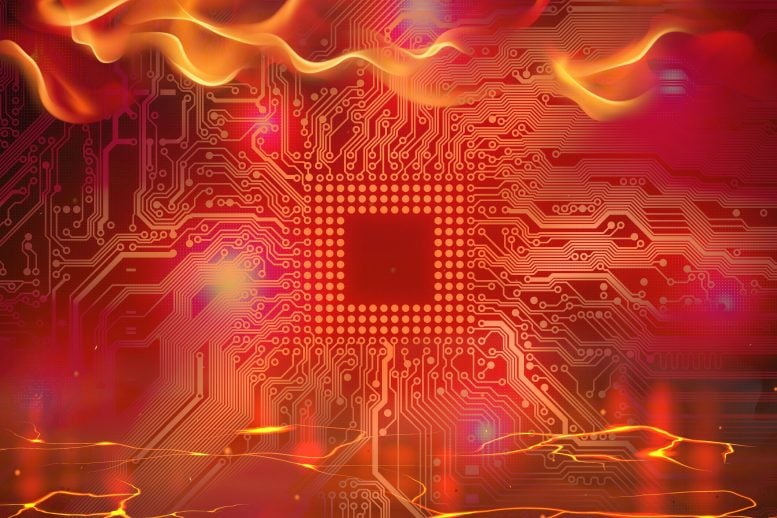
Researchers studied how temperatures up to 500 degrees Celsius would affect electronic devices made from gallium nitride, a key step in their multiyear research effort to develop electronics that can operate in extremely hot environments, like the surface of Venus. Credit: MIT News; iStock
Researchers are turning to gallium nitride for high-temperature applications such as <span class="glossaryLink" aria-describedby="tt" data-cmtooltip="
” data-gt-translate-attributes=”[{"attribute":"data-cmtooltip", "format":"html"}]” tabindex=”0″ role=”link”>Venus exploration, due to its ability to withstand temperatures above 500 degrees <span class="glossaryLink" aria-describedby="tt" data-cmtooltip="
” data-gt-translate-attributes=”[{"attribute":"data-cmtooltip", "format":"html"}]” tabindex=”0″ role=”link”>Celsius.
A recent study by <span class="glossaryLink" aria-describedby="tt" data-cmtooltip="
” data-gt-translate-attributes=”[{"attribute":"data-cmtooltip", "format":"html"}]” tabindex=”0″ role=”link”>MIT and other institutions has shown that this material, along with its ohmic contacts, remains structurally stable even at these high temperatures. The study involved building gallium nitride devices and testing them under high-temperature conditions, revealing promising results for future electronics in extreme environments.
Venus Exploration and Gallium Nitride
On the scorching surface of Venus, temperatures can climb to 480° Celsius /900° <span class="glossaryLink" aria-describedby="tt" data-cmtooltip="
” data-gt-translate-attributes=”[{"attribute":"data-cmtooltip", "format":"html"}]” tabindex=”0″ role=”link”>Fahrenheit, which is hot enough to melt lead. This makes it an inhospitable place for humans and machines alike. One reason scientists have not yet been able to send a rover to the planet’s surface is that silicon-based electronics can’t operate in such extreme temperatures for an extended period of time.
For high-temperature applications like Venus exploration, researchers have recently turned to gallium nitride, a unique material that can withstand temperatures of 500 degrees or more.
The material is already used in some terrestrial electronics, like phone chargers and cell phone towers, but scientists don’t have a good grasp of how gallium nitride devices would behave at temperatures beyond 300 degrees, which is the operational limit of conventional silicon electronics.
Research Findings on Gallium Nitride
In a new paper published in <span class="glossaryLink" aria-describedby="tt" data-cmtooltip="
” data-gt-translate-attributes=”[{"attribute":"data-cmtooltip", "format":"html"}]” tabindex=”0″ role=”link”>Applied Physics Letters, which is part of a multiyear research effort, a team of scientists from MIT and elsewhere sought to answer key questions about the material’s properties and performance at extremely high temperatures.
They studied the impact of temperature on the ohmic contacts in a gallium nitride device. Ohmic contacts are key components that connect a semiconductor device with the outside world.
The researchers found that extreme temperatures didn’t cause significant degradation to the gallium nitride material or contacts. They were surprised to see that the contacts remained structurally intact even when held at 500 degrees Celsius for 48 hours.
Future Directions in High-Temperature Electronics
Understanding how contacts perform at extreme temperatures is an important step toward the group’s next goal of developing high-performance transistors that could operate on the surface of Venus. Such transistors could also be used on Earth in electronics for applications like extracting geothermal energy or monitoring the inside of jet engines.
“Transistors are the heart of most modern electronics, but we didn’t want to jump straight to making a gallium nitride transistor because so much could go wrong. We first wanted to make sure the material and contacts could survive, and figure out how much they change as you increase the temperature. We’ll design our transistor from these basic material building blocks,” says John Niroula, an electrical engineering and computer science (EECS) graduate student and lead author of the paper.
His co-authors include Qingyun Xie PhD ’24; Mengyang Yuan PhD ’22; EECS graduate students Patrick K. Darmawi-Iskandar and Pradyot Yadav; Gillian K. Micale, a graduate student in the Department of Materials Science and Engineering; senior author Tomás Palacios, the Clarence J. LeBel Professor of EECS, director of the Microsystems Technology Laboratories, and a member of the Research Laboratory of Electronics; as well as collaborators Nitul S. Rajput of the Technology Innovation Institute of the United Arab Emirates; Siddharth Rajan of Ohio State University; Yuji Zhao of Rice University; and Nadim Chowdhury of Bangladesh University of Engineering and Technology.
Resistance and Performance Under Heat
While gallium nitride has recently attracted much attention, the material is still decades behind silicon when it comes to scientists’ understanding of how its properties change under different conditions. One such property is resistance, the flow of electrical current through a material.
A device’s overall resistance is inversely proportional to its size. But devices like <span class="glossaryLink" aria-describedby="tt" data-cmtooltip="
” data-gt-translate-attributes=”[{"attribute":"data-cmtooltip", "format":"html"}]” tabindex=”0″ role=”link”>semiconductors have contacts that connect them to other electronics. Contact resistance, which is caused by these electrical connections, remains fixed no matter the size of the device. Too much contact resistance can lead to higher power dissipation and slower operating frequencies for electronic circuits.
“Especially when you go to smaller dimensions, a device’s performance often ends up being limited by contact resistance. People have a relatively good understanding of contact resistance at room temperature, but no one has really studied what happens when you go all the way up to 500 degrees,” Niroula says.
Testing Methodologies and Results
For their study, the researchers used facilities at MIT.nano to build gallium nitride devices known as transfer length method structures, which are composed of a series of resistors. These devices enable them to measure the resistance of both the material and the contacts.
They added ohmic contacts to these devices using the two most common methods. The first involves depositing metal onto gallium nitride and heating it to 825 degrees Celsius for about 30 seconds, a process called annealing.
The second method involves removing chunks of gallium nitride and using a high-temperature technology to regrow highly doped gallium nitride in its place, a process led by Rajan and his team at Ohio State. The highly doped material contains extra electrons that can contribute to current conduction.
“The regrowth method typically leads to lower contact resistance at room temperature, but we wanted to see if these methods still work well at high temperatures,” Niroula says.
Stability and Performance at High Temperatures
They tested devices in two ways. Their collaborators at Rice University, led by Zhao, conducted short-term tests by placing devices on a hot chuck that reached 500 degrees Celsius and taking immediate resistance measurements.
At MIT, they conducted longer-term experiments by placing devices into a specialized furnace the group previously developed. They left devices inside for up to 72 hours to measure how resistance changes as a function of temperature and time.
Long-Term Stability and Improvements
Microscopy experts at MIT.nano (Aubrey N. Penn) and the Technology Innovation Institute (Nitul S. Rajput) used state-of-the-art transmission electron microscopes to see how such high temperatures affect gallium nitride and the ohmic contacts at the atomic level.
“We went in thinking the contacts or the gallium nitride material itself would degrade significantly, but we found the opposite. Contacts made with both methods seemed to be remarkably stable,” says Niroula.
While it is difficult to measure resistance at such high temperatures, their results indicate that contact resistance seems to remain constant even at temperatures of 500 degrees, for around 48 hours. And just like at room temperature, the regrowth process led to better performance.
The material did start to degrade after being in the furnace for 48 hours, but the researchers are already working to boost long-term performance. One strategy involves adding protective insulators to keep the material from being directly exposed to the high-temperature environment.
Future Prospects in Microelectronics
Moving forward, the researchers plan to use what they learned in these experiments to develop high-temperature gallium nitride transistors.
“In our group, we focus on innovative, device-level research to advance the frontiers of microelectronics, while adopting a systematic approach across the hierarchy, from the material level to the circuit level. Here, we have gone all the way down to the material level to understand things in depth. In other words, we have translated device-level advancements to circuit-level impact for high-temperature electronics, through design, modeling and complex fabrication. We are also immensely fortunate to have forged close partnerships with our longtime collaborators in this journey,” Xie says.
Reference: “High temperature stability of regrown and alloyed Ohmic contacts to AlGaN/GaN heterostructure up to 500 °C” by John Niroula, Qingyun Xie, Nitul S. Rajput, Patrick K. Darmawi-Iskandar, Sheikh Ifatur Rahman, Shisong Luo, Rafid Hassan Palash, Bejoy Sikder, Mengyang Yuan, Pradyot Yadav, Gillian K. Micale, Nadim Chowdhury, Yuji Zhao, Siddharth Rajan and Tomás Palacios, 15 May 2024, Applied Physics Letters.
DOI: 10.1063/5.0191297
This work was funded, in part, by the U.S. Air Force Office of Scientific Research, Lockheed Martin Corporation, the Semiconductor Research Corporation through the U.S. Defense Advanced Research Projects Agency, the U.S. Department of Energy, Intel Corporation, and the Bangladesh University of Engineering and Technology.
Fabrication and microscopy were conducted at MIT.nano, the Semiconductor Epitaxy and Analysis Laboratory at Ohio State University, the Center for Advanced Materials Characterization at the <span class="glossaryLink" aria-describedby="tt" data-cmtooltip="
” data-gt-translate-attributes=”[{"attribute":"data-cmtooltip", "format":"html"}]” tabindex=”0″ role=”link”>University of Oregon, and the Technology Innovation Institute of the United Arab Emirates.